Difference between revisions of "User:Debra Tabron/sandbox"
Debra Tabron (talk | contribs) |
Debra Tabron (talk | contribs) |
||
Line 1: | Line 1: | ||
− | + | N-Nitr2017osodimethylamine (NDMA) is a suspected human carcinogen that can enter water supplies from some military and industrial sources. NDMA is mobile in water, does not strongly sorb to solids or volatilize, and does not easily biodegrade. However, recent work has shown that NDMA can be effectively treated in both above ground and ''in situ'' cometabolic biological treatment systems. | |
<div style="float:right;margin:0 0 2em 2em;">__TOC__</div> | <div style="float:right;margin:0 0 2em 2em;">__TOC__</div> | ||
'''Related Article(s):''' | '''Related Article(s):''' | ||
− | *[[ | + | *[[In Situ Anaerobic Bioremediation]] |
− | *[[ | + | *[[In Situ Anaerobic Bioremediation Design Considerations]] |
+ | *[[Chlorinated Solvents]] | ||
− | |||
+ | '''CONTRIBUTOR(S):''' [[Paul Hatzinger]] | ||
'''Key Resource(s)''': | '''Key Resource(s)''': | ||
− | *[[media: | + | *[[media:2015-Hatzinger-Field_Demonstration_of_Propane_Biosparging.pdf| Field Demonstration of Propane Biosparging for "In Situ" Remediation of NDMA]]<ref name= "Hatzinger2015">Hatzinger, P.B. and Lippincott, D., 2015. Field Demonstration of Propane Biosparging for In Situ Remediation of N-Nitrosodimethylamine (NDMA) in Groundwater. ESTCP Project ER-200828 [[media:2015-Hatzinger-Field_Demonstration_of_Propane_Biosparging.pdf| Report.pdf]]</ref> |
+ | *[https://doi.org/10.1089/109287503768335896 N-nitrosodimethylamine (NDMA) as a drinking water contaminant: a review]<ref name= "Mitch2003">Mitch, W.A., Sharp, J.O., Trussell, R.R., Valentine, R.L., Alvarez-Cohen, L. and Sedlak, D.L., 2003. N-nitrosodimethylamine (NDMA) as a drinking water contaminant: a review. Environmental Engineering Science, 20(5), pp.389-404. [https://doi.org/10.1089/109287503768335896 doi: 10.1089/109287503768335896]</ref> | ||
− | == | + | ==Introduction== |
− | + | N-Nitrosodimethylamine (NDMA) is a suspected human carcinogen that has traditionally been detected in specific food products, such as cured meats (e.g., cold cuts preserved with nitrite), smoked fish, and some cheeses as well as chewing and smoking tobacco. NDMA is not commercially manufactured, but potential industrial sources include byproducts from rubber tire manufacturing, tanneries, some pesticide and dye plants, and fish processing facilities. NDMA has also been detected in groundwater at several military and aerospace sites involved in the production, testing, and/or disposal of liquid rocket propellants formulated with 1,1-dimethylhydrazine (1,1-DMH or UDMH). It is also frequently reported at ng/L concentrations in water and wastewater that has been disinfected with chloramine<ref name= "Mitch2003"/>. | |
− | + | [[File:Hatzinger1w2 Fig1.png|thumb|Figure 1. NDMA chemical structure]] | |
− | + | No Federal Maximum Contaminant Level (MCL) has been promulgated for NDMA in drinking water because it was historically thought to be an issue associated primarily with foods and tobacco, but NDMA and other nitrosamines were added by USEPA to both the Unregulated Contaminant Monitoring Rule-2 (UCMR-2) and Contaminant Candidate List-3 (CCL3). UCMR-2 requires many large water utilities to sample for the compound, and CCL3 provides a potential basis for contaminant regulation under the Safe Drinking Water Act (SDWA) based on occurrence and potential risk to the public. | |
− | |||
− | + | Among the states, California has established a public health goal of 3 ng/L and Massachusetts has set a regulatory standard of 10 ng/L in drinking water. These stringent requirements reflect the carcinogenicity of NDMA which, based on USEPA risk assessments, poses an increased cancer risk of one cancer per million at a drinking water concentration of only 0.7 ng/L<ref name= "USEPA2014TFS">USEPA. 2014. Technical Fact Sheet - N-Nitroso-dimethylamine. USEPA Office of Solid Waste and Emergency Response. EPA 505-F-14-005 [[media:2014-USEPA-N-Nitrosodimethylamine_fact_sheet.pdf| Report.pdf]]</ref>. | |
− | + | ==Physical and Chemical Properties== | |
+ | NDMA, in its pure form, is a yellow liquid with a density of 1.0059 g/ml and molecular weight of 74.1 g/mol<ref name= "USEPA2014TFS"/>. It is miscible in water, relatively non-volatile and sorbs poorly to organic carbon, allowing it to readily migrate with groundwater. While NDMA is susceptible to photolysis, it is relatively recalcitrant in groundwater under both oxic and anoxic conditions, and forms large plumes at sites with concentrated sources (e.g., rocket motor test sites). | ||
− | [[File: | + | ==Remediation== |
− | + | [[File:Hatzinger1w2 Fig2.png|thumb|Figure 2. Field-Scale Propane-Fed FBR.]] | |
+ | [[File:Hatzinger1w2 Fig3.png|thumb|Figure 3. Layout of biosparging system<ref name= "Hatzinger2015"/>.]] | ||
+ | [[File:Hatzinger1w2 Fig4.png|thumb|Figure 4. Field-Scale "In Situ" Propane Biosparging System]] | ||
− | + | NDMA is typically removed from groundwater by extracting the contaminated groundwater followed by surface treatment with ultraviolet radiation (UV). NDMA decomposes in UV light in the 225 - 250 nm wavelength range. The primary products are dimethylamine and nitrite<ref>Stefan, M.I. and Bolton, J.R., 2002. UV direct photolysis of N‐nitrosodimethylamine (NDMA): Kinetic and product study. Helvetica Chimica Acta, 85(5), pp.1416-1426. [https://doi.org/10.1002/1522-2675(200205)85:5<1416::AID-HLCA1416>3.0.CO;2-I doi: 10.1002/1522-2675(200205)85:5<1416::AID-HLCA1416>3.0.CO;2-I]</ref>. UV treatment can reduce NDMA concentrations to low ng/L levels, but is expensive due to the high energy input required. In potable water treatment systems, the UV dosage for NDMA removal can be a factor of ten greater than the dosage required to achieve equivalent virus removal<ref name= "Mitch2003"/>. | |
− | + | Cometabolic biological treatment is an emerging technology for above ground and ''in situ'' treatment of NDMA. A variety of propane-oxidizing bacteria (propanotrophs) are capable of degrading NDMA<ref>Sharp, J.O., Sales, C.M., LeBlanc, J.C., Liu, J., Wood, T.K., Eltis, L.D., Mohn, W.W. and Alvarez-Cohen, L., 2007. An inducible propane monooxygenase is responsible for N-nitrosodimethylamine degradation by Rhodococcus sp. strain RHA1. Applied and Environmental Microbiology, 73(21), pp.6930-6938. [https://doi.org/10.1128/aem.01697-07 doi: 10.1128/AEM.01697-07]</ref><ref name= "Fournier2009">Fournier, D., Hawari, J., Halasz, A., Streger, S.H., McClay, K.R., Masuda, H. and Hatzinger, P.B., 2009. Aerobic biodegradation of N-nitrosodimethylamine by the propanotroph Rhodococcus ruber ENV425. Applied and environmental microbiology, 75(15), pp.5088-5093. [https://doi.org/10.1128/aem.00418-09 doi: 10.1128/AEM.00418-09]</ref><ref name= "Weidhaas2012">Weidhaas, J.L., Zigmond, M.J. and Dupont, R.R., 2012. Aerobic biotransformation of N-nitrosodimethylamine and N-nitrodimethylamine by benzene-, butane-, methane-, propane-, and toluene-fed cultures. Bioremediation Journal, 16(2), pp.74-85. [https://doi.org/10.1080/10889868.2012.665961 doi: 10.1080/10889868.2012.665961]</ref>. When fed propane, these organisms produce a broad specificity monooxygenase enzyme (propane monooxygenase; PrMO) that cometabolically transforms NDMA producing methylamine, nitric oxide, nitrite, nitrate, and formate. A major advantage of cometabolic degradation is that the microorganisms grow on the primary substrate (propane) allowing NDMA to be reduced to ultra-low concentrations. For example, the bacterium ''Rhodococcus ruber'' ENV425 degraded NDMA from concentrations as high as 80 g/L to < 10 ng/L in batch culture<ref name= "Fournier2009"/>, and in a membrane bioreactor<ref>Hatzinger, P.B., Condee, C., McClay, K.R. and Togna, A.P., 2011. Aerobic treatment of N-nitrosodimethylamine in a propane-fed membrane bioreactor. Water research, 45(1), pp.254-262. [https://doi.org/10.1016/j.watres.2010.07.056 doi: 10.1016/j.watres.2010.07.056]</ref>. A mixed culture grown on propane also degraded NDMA to < 10 ng/L<ref name= "Weidhaas2012"/>. | |
− | = | + | NDMA impacted groundwater has been successfully treated in full-scale biological fluidized bed reactors (BFBR)<ref name= "Hatzinger2017">Hatzinger, P.B., Lewis, C. and Webster, T.S., 2017. Biological treatment of N-nitrosodimethylamine (NDMA) and N-nitrodimethylamine (NTDMA) in a field-scale fluidized bed bioreactor. Water Research, 126, pp.361-371. [https://doi.org/10.1016/j.watres.2017.09.040 doi: 10.1016/j.watres.2017.09.040]</ref>. Full-scale BFBRs are currently used for treatment of nitrate, perchlorate, selenium, volatile organic compounds (VOCs), and chlorinated solvents<ref>Hatzinger, P.B., 2005. Perchlorate biodegradation for water treatment. Environ. Sci. Technol., 2005, 39 (11), pp 239A–247A [https://doi.org/10.1021/es053280x doi: 10.1021/es053280x]</ref><ref>Sutton, P.M. and Mishra, P.N., 1994. Activated carbon based biological fluidized beds for contaminated water and wastewater treatment: a state-of-the-art review. Water Science and Technology, 29(10-11), pp.309-317. [https://doi.org/10.2166/wst.1994.0774 doi: 10.2166/wst.1994.0774]</ref><ref name= "Webser2009">Webster, T.S., Guarini, W.J. and Wong, H.S., 2009. Fluidized bed bioreactor treatment of perchlorate–laden groundwater to potable standards. Journal‐American Water Works Association, 101(5), pp.137-151. [https://doi.org/10.1002/j.1551-8833.2009.tb09896.x doi: 10.1002/j.1551-8833.2009.tb09896.x]</ref>. Fluidized bed reactors have also been utilized in California as part of a drinking water treatment system<ref name= "Webser2009"/><ref>Webster, T.S. and Litchfield, M.H., 2017. Full‐Scale Biological Treatment of Nitrate and Perchlorate for Potable Water Production. Journal‐American Water Works Association, 109(5), pp.30-40. [https://doi.org/10.5942/jawwa.2017.109.0065 doi: 0.5942/jawwa.2017.109.0065]</ref>. |
− | |||
− | + | At a former rocket test site, a BFBR was operated at a fluidization flow of ~ 9 liters per minute (LPM) and received propane, oxygen, and inorganic nutrients in the feed, along with an influent concentration of ~1,000 ng/L NDMA (Fig. 2). At an average hydraulic residence time (HRT) of 10 min, NDMA was reduced to less than 10 ng/L. At a 20 min HRT, NDMA was reduced to below the site discharge limit of 4.2 ng/L. The BFBR system is highly resilient to upsets by trace co-contaminants such as trichloroethene (TCE) and Freon 11<ref name= "Hatzinger2017"/>. | |
− | + | ''In situ'' propane amendment (Fig. 3) was also effective for treating NDMA in groundwater at the Aerojet Superfund Site in Rancho Cordova, CA. The propane biosparging demonstration (Fig. 4) was conducted downgradient of the liquid rocket engine test area where NDMA concentrations in groundwater ranged from 2,000 to 30,000 ng/L. The propane and air biosparging system removed 99.7 % to > 99.9 % of the initial NDMA to levels as low 3 ng/L. Propane biosparging systems are expected to be less expensive to install and operate than conventional pump-and-treat systems<ref name= "Hatzinger2015"/>. | |
− | + | ||
− | + | ==Summary== | |
− | + | N-Nitrosodimethylamine (NDMA) is a suspected human carcinogen often found in tobacco and in certain foods including cured meats, smoked fish and some cheeses. While not commercially manufactured, NDMA can occur when water is disinfected with chloramine or from industrial and military sources. NDMA does not readily volatilize, sorb to solid material, or biodegrade so it is mobile in groundwater. While NDMA can be degraded to low levels with ultraviolet radiation (UV), energy costs are high. Cometabolic biological treatment is an emerging technology that has been demonstrated to be effective for both above ground and ''in situ'' treatment of NDMA. | |
− | |||
− | |||
− | |||
− | |||
− | |||
− | |||
− | |||
− | |||
− | |||
− | |||
− | |||
− | |||
− | |||
− | |||
− | |||
− | |||
− | == | ||
− | |||
− | |||
− | |||
− | |||
− | |||
− | |||
− | |||
− | |||
− | |||
==References== | ==References== | ||
Line 72: | Line 49: | ||
==See Also== | ==See Also== | ||
− |
Revision as of 17:54, 11 February 2019
N-Nitr2017osodimethylamine (NDMA) is a suspected human carcinogen that can enter water supplies from some military and industrial sources. NDMA is mobile in water, does not strongly sorb to solids or volatilize, and does not easily biodegrade. However, recent work has shown that NDMA can be effectively treated in both above ground and in situ cometabolic biological treatment systems.
Related Article(s):
- In Situ Anaerobic Bioremediation
- In Situ Anaerobic Bioremediation Design Considerations
- Chlorinated Solvents
CONTRIBUTOR(S): Paul Hatzinger
Key Resource(s):
- Field Demonstration of Propane Biosparging for "In Situ" Remediation of NDMA[1]
- N-nitrosodimethylamine (NDMA) as a drinking water contaminant: a review[2]
Introduction
N-Nitrosodimethylamine (NDMA) is a suspected human carcinogen that has traditionally been detected in specific food products, such as cured meats (e.g., cold cuts preserved with nitrite), smoked fish, and some cheeses as well as chewing and smoking tobacco. NDMA is not commercially manufactured, but potential industrial sources include byproducts from rubber tire manufacturing, tanneries, some pesticide and dye plants, and fish processing facilities. NDMA has also been detected in groundwater at several military and aerospace sites involved in the production, testing, and/or disposal of liquid rocket propellants formulated with 1,1-dimethylhydrazine (1,1-DMH or UDMH). It is also frequently reported at ng/L concentrations in water and wastewater that has been disinfected with chloramine[2].
No Federal Maximum Contaminant Level (MCL) has been promulgated for NDMA in drinking water because it was historically thought to be an issue associated primarily with foods and tobacco, but NDMA and other nitrosamines were added by USEPA to both the Unregulated Contaminant Monitoring Rule-2 (UCMR-2) and Contaminant Candidate List-3 (CCL3). UCMR-2 requires many large water utilities to sample for the compound, and CCL3 provides a potential basis for contaminant regulation under the Safe Drinking Water Act (SDWA) based on occurrence and potential risk to the public.
Among the states, California has established a public health goal of 3 ng/L and Massachusetts has set a regulatory standard of 10 ng/L in drinking water. These stringent requirements reflect the carcinogenicity of NDMA which, based on USEPA risk assessments, poses an increased cancer risk of one cancer per million at a drinking water concentration of only 0.7 ng/L[3].
Physical and Chemical Properties
NDMA, in its pure form, is a yellow liquid with a density of 1.0059 g/ml and molecular weight of 74.1 g/mol[3]. It is miscible in water, relatively non-volatile and sorbs poorly to organic carbon, allowing it to readily migrate with groundwater. While NDMA is susceptible to photolysis, it is relatively recalcitrant in groundwater under both oxic and anoxic conditions, and forms large plumes at sites with concentrated sources (e.g., rocket motor test sites).
Remediation
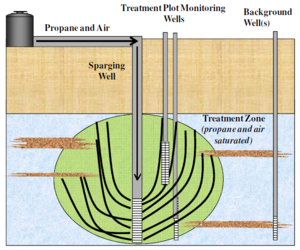
NDMA is typically removed from groundwater by extracting the contaminated groundwater followed by surface treatment with ultraviolet radiation (UV). NDMA decomposes in UV light in the 225 - 250 nm wavelength range. The primary products are dimethylamine and nitrite[4]. UV treatment can reduce NDMA concentrations to low ng/L levels, but is expensive due to the high energy input required. In potable water treatment systems, the UV dosage for NDMA removal can be a factor of ten greater than the dosage required to achieve equivalent virus removal[2].
Cometabolic biological treatment is an emerging technology for above ground and in situ treatment of NDMA. A variety of propane-oxidizing bacteria (propanotrophs) are capable of degrading NDMA[5][6][7]. When fed propane, these organisms produce a broad specificity monooxygenase enzyme (propane monooxygenase; PrMO) that cometabolically transforms NDMA producing methylamine, nitric oxide, nitrite, nitrate, and formate. A major advantage of cometabolic degradation is that the microorganisms grow on the primary substrate (propane) allowing NDMA to be reduced to ultra-low concentrations. For example, the bacterium Rhodococcus ruber ENV425 degraded NDMA from concentrations as high as 80 g/L to < 10 ng/L in batch culture[6], and in a membrane bioreactor[8]. A mixed culture grown on propane also degraded NDMA to < 10 ng/L[7].
NDMA impacted groundwater has been successfully treated in full-scale biological fluidized bed reactors (BFBR)[9]. Full-scale BFBRs are currently used for treatment of nitrate, perchlorate, selenium, volatile organic compounds (VOCs), and chlorinated solvents[10][11][12]. Fluidized bed reactors have also been utilized in California as part of a drinking water treatment system[12][13].
At a former rocket test site, a BFBR was operated at a fluidization flow of ~ 9 liters per minute (LPM) and received propane, oxygen, and inorganic nutrients in the feed, along with an influent concentration of ~1,000 ng/L NDMA (Fig. 2). At an average hydraulic residence time (HRT) of 10 min, NDMA was reduced to less than 10 ng/L. At a 20 min HRT, NDMA was reduced to below the site discharge limit of 4.2 ng/L. The BFBR system is highly resilient to upsets by trace co-contaminants such as trichloroethene (TCE) and Freon 11[9].
In situ propane amendment (Fig. 3) was also effective for treating NDMA in groundwater at the Aerojet Superfund Site in Rancho Cordova, CA. The propane biosparging demonstration (Fig. 4) was conducted downgradient of the liquid rocket engine test area where NDMA concentrations in groundwater ranged from 2,000 to 30,000 ng/L. The propane and air biosparging system removed 99.7 % to > 99.9 % of the initial NDMA to levels as low 3 ng/L. Propane biosparging systems are expected to be less expensive to install and operate than conventional pump-and-treat systems[1].
Summary
N-Nitrosodimethylamine (NDMA) is a suspected human carcinogen often found in tobacco and in certain foods including cured meats, smoked fish and some cheeses. While not commercially manufactured, NDMA can occur when water is disinfected with chloramine or from industrial and military sources. NDMA does not readily volatilize, sorb to solid material, or biodegrade so it is mobile in groundwater. While NDMA can be degraded to low levels with ultraviolet radiation (UV), energy costs are high. Cometabolic biological treatment is an emerging technology that has been demonstrated to be effective for both above ground and in situ treatment of NDMA.
References
- ^ 1.0 1.1 1.2 Hatzinger, P.B. and Lippincott, D., 2015. Field Demonstration of Propane Biosparging for In Situ Remediation of N-Nitrosodimethylamine (NDMA) in Groundwater. ESTCP Project ER-200828 Report.pdf
- ^ 2.0 2.1 2.2 Mitch, W.A., Sharp, J.O., Trussell, R.R., Valentine, R.L., Alvarez-Cohen, L. and Sedlak, D.L., 2003. N-nitrosodimethylamine (NDMA) as a drinking water contaminant: a review. Environmental Engineering Science, 20(5), pp.389-404. doi: 10.1089/109287503768335896
- ^ 3.0 3.1 USEPA. 2014. Technical Fact Sheet - N-Nitroso-dimethylamine. USEPA Office of Solid Waste and Emergency Response. EPA 505-F-14-005 Report.pdf
- ^ Stefan, M.I. and Bolton, J.R., 2002. UV direct photolysis of N‐nitrosodimethylamine (NDMA): Kinetic and product study. Helvetica Chimica Acta, 85(5), pp.1416-1426. <1416::AID-HLCA1416>3.0.CO;2-I doi: 10.1002/1522-2675(200205)85:5<1416::AID-HLCA1416>3.0.CO;2-I
- ^ Sharp, J.O., Sales, C.M., LeBlanc, J.C., Liu, J., Wood, T.K., Eltis, L.D., Mohn, W.W. and Alvarez-Cohen, L., 2007. An inducible propane monooxygenase is responsible for N-nitrosodimethylamine degradation by Rhodococcus sp. strain RHA1. Applied and Environmental Microbiology, 73(21), pp.6930-6938. doi: 10.1128/AEM.01697-07
- ^ 6.0 6.1 Fournier, D., Hawari, J., Halasz, A., Streger, S.H., McClay, K.R., Masuda, H. and Hatzinger, P.B., 2009. Aerobic biodegradation of N-nitrosodimethylamine by the propanotroph Rhodococcus ruber ENV425. Applied and environmental microbiology, 75(15), pp.5088-5093. doi: 10.1128/AEM.00418-09
- ^ 7.0 7.1 Weidhaas, J.L., Zigmond, M.J. and Dupont, R.R., 2012. Aerobic biotransformation of N-nitrosodimethylamine and N-nitrodimethylamine by benzene-, butane-, methane-, propane-, and toluene-fed cultures. Bioremediation Journal, 16(2), pp.74-85. doi: 10.1080/10889868.2012.665961
- ^ Hatzinger, P.B., Condee, C., McClay, K.R. and Togna, A.P., 2011. Aerobic treatment of N-nitrosodimethylamine in a propane-fed membrane bioreactor. Water research, 45(1), pp.254-262. doi: 10.1016/j.watres.2010.07.056
- ^ 9.0 9.1 Hatzinger, P.B., Lewis, C. and Webster, T.S., 2017. Biological treatment of N-nitrosodimethylamine (NDMA) and N-nitrodimethylamine (NTDMA) in a field-scale fluidized bed bioreactor. Water Research, 126, pp.361-371. doi: 10.1016/j.watres.2017.09.040
- ^ Hatzinger, P.B., 2005. Perchlorate biodegradation for water treatment. Environ. Sci. Technol., 2005, 39 (11), pp 239A–247A doi: 10.1021/es053280x
- ^ Sutton, P.M. and Mishra, P.N., 1994. Activated carbon based biological fluidized beds for contaminated water and wastewater treatment: a state-of-the-art review. Water Science and Technology, 29(10-11), pp.309-317. doi: 10.2166/wst.1994.0774
- ^ 12.0 12.1 Webster, T.S., Guarini, W.J. and Wong, H.S., 2009. Fluidized bed bioreactor treatment of perchlorate–laden groundwater to potable standards. Journal‐American Water Works Association, 101(5), pp.137-151. doi: 10.1002/j.1551-8833.2009.tb09896.x
- ^ Webster, T.S. and Litchfield, M.H., 2017. Full‐Scale Biological Treatment of Nitrate and Perchlorate for Potable Water Production. Journal‐American Water Works Association, 109(5), pp.30-40. doi: 0.5942/jawwa.2017.109.0065